Chapter 1: How Science Works
Chapter 2
Early Astronomy
- The Night Sky
- Motions in the Sky
- Navigation
- Constellations and Seasons
- Cause of the Seasons
- The Magnitude System
- Angular Size and Linear Size
- Phases of the Moon
- Eclipses
- Auroras
- Dividing Time
- Solar and Lunar Calendars
- History of Astronomy
- Stonehenge
- Ancient Observatories
- Counting and Measurement
- Astrology
- Greek Astronomy
- Aristotle and Geocentric Cosmology
- Aristarchus and Heliocentric Cosmology
- The Dark Ages
- Arab Astronomy
- Indian Astronomy
- Chinese Astronomy
- Mayan Astronomy
- Questions
Chapter 3
The Copernican Revolution
- Ptolemy and the Geocentric Model
- The Renaissance
- Copernicus and the Heliocentric Model
- Tycho Brahe
- Johannes Kepler
- Elliptical Orbits
- Kepler's Laws
- Galileo Galilei
- The Trial of Galileo
- Isaac Newton
- Newton's Law of Gravity
- The Plurality of Worlds
- The Birth of Modern Science
- Layout of the Solar System
- Scale of the Solar System
- The Idea of Space Exploration
- Orbits
- History of Space Exploration
- Moon Landings
- International Space Station
- Manned versus Robotic Missions
- Commercial Space Flight
- Future of Space Exploration
- Living in Space
- Moon, Mars, and Beyond
- Societies in Space
- Questions
Chapter 4
Matter and Energy in the Universe
- Matter and Energy
- Rutherford and Atomic Structure
- Early Greek Physics
- Dalton and Atoms
- The Periodic Table
- Structure of the Atom
- Energy
- Heat and Temperature
- Potential and Kinetic Energy
- Conservation of Energy
- Velocity of Gas Particles
- States of Matter
- Thermodynamics
- Entropy
- Laws of Thermodynamics
- Heat Transfer
- Thermal Radiation
- Wien's Law
- Radiation from Planets and Stars
- Internal Heat in Planets and Stars
- Periodic Processes
- Random Processes
- Questions
Chapter 5
The Earth-Moon System
- Earth and Moon
- Early Estimates of Earth's Age
- How the Earth Cooled
- Ages Using Radioactivity
- Radioactive Half-Life
- Ages of the Earth and Moon
- Geological Activity
- Internal Structure of the Earth and Moon
- Basic Rock Types
- Layers of the Earth and Moon
- Origin of Water on Earth
- The Evolving Earth
- Plate Tectonics
- Volcanoes
- Geological Processes
- Impact Craters
- The Geological Timescale
- Mass Extinctions
- Evolution and the Cosmic Environment
- Earth's Atmosphere and Oceans
- Weather Circulation
- Environmental Change on Earth
- The Earth-Moon System
- Geological History of the Moon
- Tidal Forces
- Effects of Tidal Forces
- Historical Studies of the Moon
- Lunar Surface
- Ice on the Moon
- Origin of the Moon
- Humans on the Moon
- Questions
Chapter 6
The Terrestrial Planets
- Studying Other Planets
- The Planets
- The Terrestrial Planets
- Mercury
- Mercury's Orbit
- Mercury's Surface
- Venus
- Volcanism on Venus
- Venus and the Greenhouse Effect
- Tectonics on Venus
- Exploring Venus
- Mars in Myth and Legend
- Early Studies of Mars
- Mars Close-Up
- Modern Views of Mars
- Missions to Mars
- Geology of Mars
- Water on Mars
- Polar Caps of Mars
- Climate Change on Mars
- Terraforming Mars
- Life on Mars
- The Moons of Mars
- Martian Meteorites
- Comparative Planetology
- Incidence of Craters
- Counting Craters
- Counting Statistics
- Internal Heat and Geological Activity
- Magnetic Fields of the Terrestrial Planets
- Mountains and Rifts
- Radar Studies of Planetary Surfaces
- Laser Ranging and Altimetry
- Gravity and Atmospheres
- Normal Atmospheric Composition
- The Significance of Oxygen
- Questions
Chapter 7
The Giant Planets and Their Moons
- The Gas Giant Planets
- Atmospheres of the Gas Giant Planets
- Clouds and Weather on Gas Giant Planets
- Internal Structure of the Gas Giant Planets
- Thermal Radiation from Gas Giant Planets
- Life on Gas Giant Planets?
- Why Giant Planets are Giant
- Gas Laws
- Ring Systems of the Giant Planets
- Structure Within Ring Systems
- The Origin of Ring Particles
- The Roche Limit
- Resonance and Harmonics
- Tidal Forces in the Solar System
- Moons of Gas Giant Planets
- Geology of Large Moons
- The Voyager Missions
- Jupiter
- Jupiter's Galilean Moons
- Jupiter's Ganymede
- Jupiter's Europa
- Jupiter's Callisto
- Jupiter's Io
- Volcanoes on Io
- Saturn
- Cassini Mission to Saturn
- Saturn's Titan
- Saturn's Enceladus
- Discovery of Uranus and Neptune
- Uranus
- Uranus' Miranda
- Neptune
- Neptune's Triton
- Pluto
- The Discovery of Pluto
- Pluto as a Dwarf Planet
- Dwarf Planets
- Questions
Chapter 8
Interplanetary Bodies
- Interplanetary Bodies
- Comets
- Early Observations of Comets
- Structure of the Comet Nucleus
- Comet Chemistry
- Oort Cloud and Kuiper Belt
- Kuiper Belt
- Comet Orbits
- Life Story of Comets
- The Largest Kuiper Belt Objects
- Meteors and Meteor Showers
- Gravitational Perturbations
- Asteroids
- Surveys for Earth Crossing Asteroids
- Asteroid Shapes
- Composition of Asteroids
- Introduction to Meteorites
- Origin of Meteorites
- Types of Meteorites
- The Tunguska Event
- The Threat from Space
- Probability and Impacts
- Impact on Jupiter
- Interplanetary Opportunity
- Questions
Chapter 9
Planet Formation and Exoplanets
- Formation of the Solar System
- Early History of the Solar System
- Conservation of Angular Momentum
- Angular Momentum in a Collapsing Cloud
- Helmholtz Contraction
- Safronov and Planet Formation
- Collapse of the Solar Nebula
- Why the Solar System Collapsed
- From Planetesimals to Planets
- Accretion and Solar System Bodies
- Differentiation
- Planetary Magnetic Fields
- The Origin of Satellites
- Solar System Debris and Formation
- Gradual Evolution and a Few Catastrophies
- Chaos and Determinism
- Extrasolar Planets
- Discoveries of Exoplanets
- Doppler Detection of Exoplanets
- Transit Detection of Exoplanets
- The Kepler Mission
- Direct Detection of Exoplanets
- Properties of Exoplanets
- Implications of Exoplanet Surveys
- Future Detection of Exoplanets
- Questions
Chapter 10
Detecting Radiation from Space
- Observing the Universe
- Radiation and the Universe
- The Nature of Light
- The Electromagnetic Spectrum
- Properties of Waves
- Waves and Particles
- How Radiation Travels
- Properties of Electromagnetic Radiation
- The Doppler Effect
- Invisible Radiation
- Thermal Spectra
- The Quantum Theory
- The Uncertainty Principle
- Spectral Lines
- Emission Lines and Bands
- Absorption and Emission Spectra
- Kirchoff's Laws
- Astronomical Detection of Radiation
- The Telescope
- Optical Telescopes
- Optical Detectors
- Adaptive Optics
- Image Processing
- Digital Information
- Radio Telescopes
- Telescopes in Space
- Hubble Space Telescope
- Interferometry
- Collecting Area and Resolution
- Frontier Observatories
- Questions
Chapter 11
Our Sun: The Nearest Star
- The Sun
- The Nearest Star
- Properties of the Sun
- Kelvin and the Sun's Age
- The Sun's Composition
- Energy From Atomic Nuclei
- Mass-Energy Conversion
- Examples of Mass-Energy Conversion
- Energy From Nuclear Fission
- Energy From Nuclear Fusion
- Nuclear Reactions in the Sun
- The Sun's Interior
- Energy Flow in the Sun
- Collisions and Opacity
- Solar Neutrinos
- Solar Oscillations
- The Sun's Atmosphere
- Solar Chromosphere and Corona
- Sunspots
- The Solar Cycle
- The Solar Wind
- Effects of the Sun on the Earth
- Cosmic Energy Sources
- Questions
Chapter 12
Properties of Stars
- Stars
- Star Names
- Star Properties
- The Distance to Stars
- Apparent Brightness
- Absolute Brightness
- Measuring Star Distances
- Stellar Parallax
- Spectra of Stars
- Spectral Classification
- Temperature and Spectral Class
- Stellar Composition
- Stellar Motion
- Stellar Luminosity
- The Size of Stars
- Stefan-Boltzmann Law
- Stellar Mass
- Hydrostatic Equilibrium
- Stellar Classification
- The Hertzsprung-Russell Diagram
- Volume and Brightness Selected Samples
- Stars of Different Sizes
- Understanding the Main Sequence
- Stellar Structure
- Stellar Evolution
- Questions
Chapter 13
Star Birth and Death
- Star Birth and Death
- Understanding Star Birth and Death
- Cosmic Abundance of Elements
- Star Formation
- Molecular Clouds
- Young Stars
- T Tauri Stars
- Mass Limits for Stars
- Brown Dwarfs
- Young Star Clusters
- Cauldron of the Elements
- Main Sequence Stars
- Nuclear Reactions in Main Sequence Stars
- Main Sequence Lifetimes
- Evolved Stars
- Cycles of Star Life and Death
- The Creation of Heavy Elements
- Red Giants
- Horizontal Branch and Asymptotic Giant Branch Stars
- Variable Stars
- Magnetic Stars
- Stellar Mass Loss
- White Dwarfs
- Supernovae
- Seeing the Death of a Star
- Supernova 1987A
- Neutron Stars and Pulsars
- Special Theory of Relativity
- General Theory of Relativity
- Black Holes
- Properties of Black Holes
- Questions
Chapter 14
The Milky Way
- The Distribution of Stars in Space
- Stellar Companions
- Binary Star Systems
- Binary and Multiple Stars
- Mass Transfer in Binaries
- Binaries and Stellar Mass
- Nova and Supernova
- Exotic Binary Systems
- Gamma Ray Bursts
- How Multiple Stars Form
- Environments of Stars
- The Interstellar Medium
- Effects of Interstellar Material on Starlight
- Structure of the Interstellar Medium
- Dust Extinction and Reddening
- Groups of Stars
- Open Star Clusters
- Globular Star Clusters
- Distances to Groups of Stars
- Ages of Groups of Stars
- Layout of the Milky Way
- William Herschel
- Isotropy and Anisotropy
- Mapping the Milky Way
- Questions
Chapter 15
Galaxies
- The Milky Way Galaxy
- Mapping the Galaxy Disk
- Spiral Structure in Galaxies
- Mass of the Milky Way
- Dark Matter in the Milky Way
- Galaxy Mass
- The Galactic Center
- Black Hole in the Galactic Center
- Stellar Populations
- Formation of the Milky Way
- Galaxies
- The Shapley-Curtis Debate
- Edwin Hubble
- Distances to Galaxies
- Classifying Galaxies
- Spiral Galaxies
- Elliptical Galaxies
- Lenticular Galaxies
- Dwarf and Irregular Galaxies
- Overview of Galaxy Structures
- The Local Group
- Light Travel Time
- Galaxy Size and Luminosity
- Mass to Light Ratios
- Dark Matter in Galaxies
- Gravity of Many Bodies
- Galaxy Evolution
- Galaxy Interactions
- Galaxy Formation
- Questions
Chapter 16
The Expanding Universe
- Galaxy Redshifts
- The Expanding Universe
- Cosmological Redshifts
- The Hubble Relation
- Relating Redshift and Distance
- Galaxy Distance Indicators
- Size and Age of the Universe
- The Hubble Constant
- Large Scale Structure
- Galaxy Clustering
- Clusters of Galaxies
- Overview of Large Scale Structure
- Dark Matter on the Largest Scales
- The Most Distant Galaxies
- Black Holes in Nearby Galaxies
- Active Galaxies
- Radio Galaxies
- The Discovery of Quasars
- Quasars
- Types of Gravitational Lensing
- Properties of Quasars
- The Quasar Power Source
- Quasars as Probes of the Universe
- Star Formation History of the Universe
- Expansion History of the Universe
- Questions
Chapter 17
Cosmology
- Cosmology
- Early Cosmologies
- Relativity and Cosmology
- The Big Bang Model
- The Cosmological Principle
- Universal Expansion
- Cosmic Nucleosynthesis
- Cosmic Microwave Background Radiation
- Discovery of the Microwave Background Radiation
- Measuring Space Curvature
- Cosmic Evolution
- Evolution of Structure
- Mean Cosmic Density
- Critical Density
- Dark Matter and Dark Energy
- Age of the Universe
- Precision Cosmology
- The Future of the Contents of the Universe
- Fate of the Universe
- Alternatives to the Big Bang Model
- Space-Time
- Particles and Radiation
- The Very Early Universe
- Mass and Energy in the Early Universe
- Matter and Antimatter
- The Forces of Nature
- Fine-Tuning in Cosmology
- The Anthropic Principle in Cosmology
- String Theory and Cosmology
- The Multiverse
- The Limits of Knowledge
- Questions
Chapter 18
Life On Earth
- Nature of Life
- Chemistry of Life
- Molecules of Life
- The Origin of Life on Earth
- Origin of Complex Molecules
- Miller-Urey Experiment
- Pre-RNA World
- RNA World
- From Molecules to Cells
- Metabolism
- Anaerobes
- Extremophiles
- Thermophiles
- Psychrophiles
- Xerophiles
- Halophiles
- Barophiles
- Acidophiles
- Alkaliphiles
- Radiation Resistant Biology
- Importance of Water for Life
- Hydrothermal Systems
- Silicon Versus Carbon
- DNA and Heredity
- Life as Digital Information
- Synthetic Biology
- Life in a Computer
- Natural Selection
- Tree Of Life
- Evolution and Intelligence
- Culture and Technology
- The Gaia Hypothesis
- Life and the Cosmic Environment
Chapter 19
Life in the Universe
- Life in the Universe
- Astrobiology
- Life Beyond Earth
- Sites for Life
- Complex Molecules in Space
- Life in the Solar System
- Lowell and Canals on Mars
- Implications of Life on Mars
- Extreme Environments in the Solar System
- Rare Earth Hypothesis
- Are We Alone?
- Unidentified Flying Objects or UFOs
- The Search for Extraterrestrial Intelligence
- The Drake Equation
- The History of SETI
- Recent SETI Projects
- Recognizing a Message
- The Best Way to Communicate
- The Fermi Question
- The Anthropic Principle
- Where Are They?
Case Study of Life on Mars
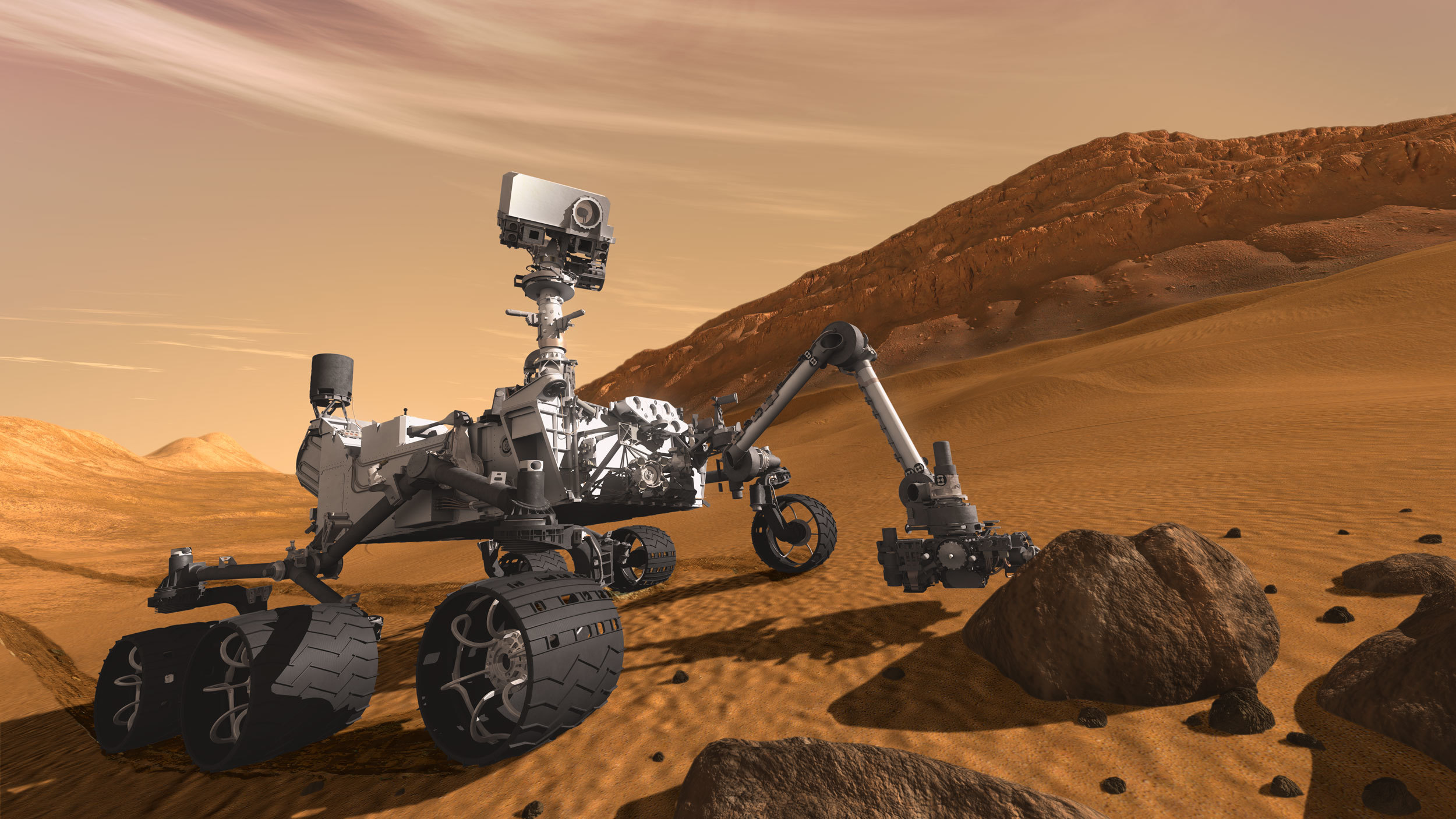
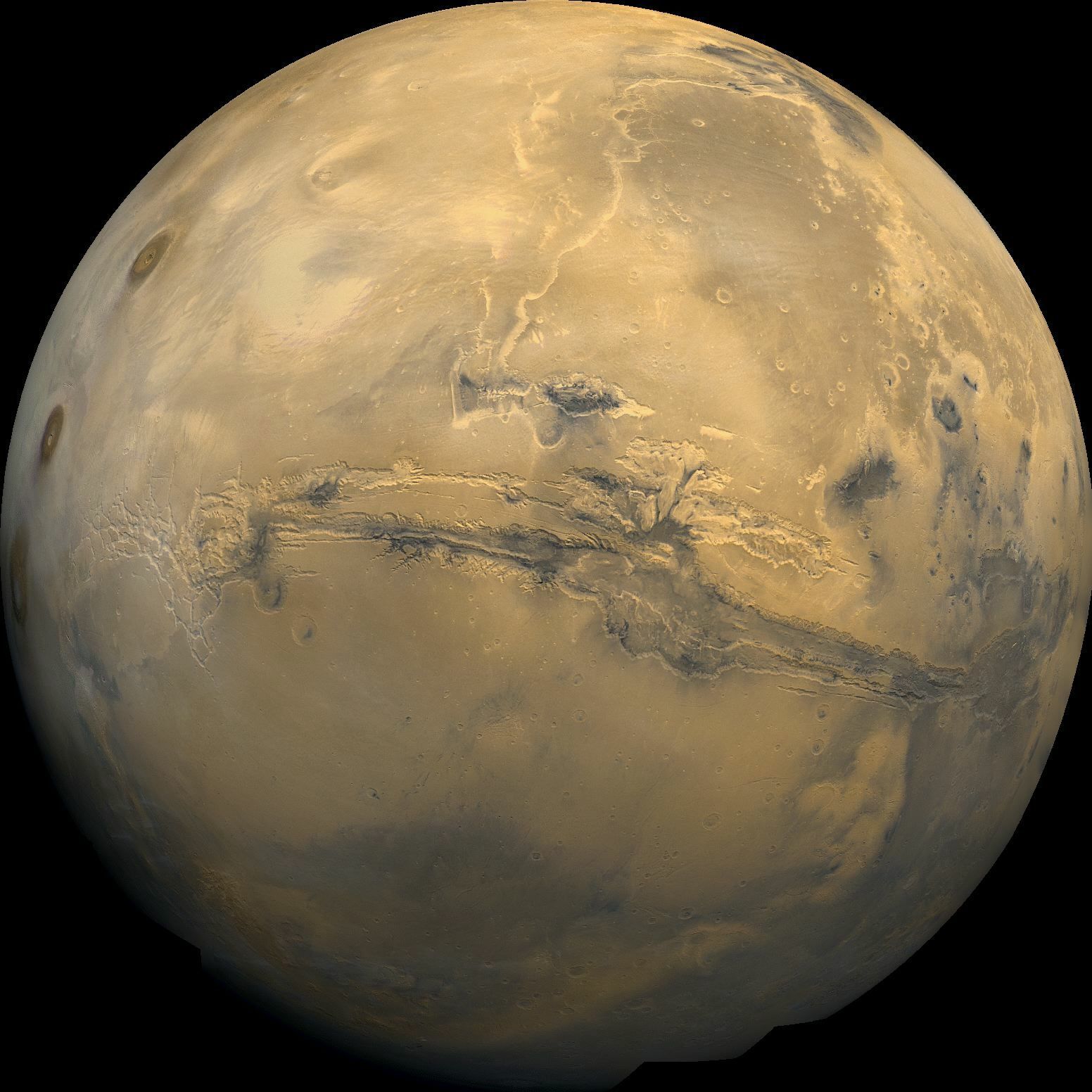
For over 100 years, one of the greatest mysteries in Solar System science has remained: Is there life on Mars? While several tantalizing results hint at the possibility of microbial life, no incontrovertible evidence exists. While we can't answer this profound question definitively, modern evidence is at least making it clear life could exist in the Martian environment. Most astrobiologists agree that for life to exist a liquid must be present to catalyze reactions. We now know that frozen water exists on the Martian polar caps, and we believe that in the past, surface flooding carved many of the chasms and canyons that cut across the Martian surface. If ancient Mars had liquid water, did life arise there? If not, why not? If it did, how advanced did it get? Where is it today? Did it become extinct? Or is it somewhere out of sight? The most recent two Mars rovers, Curiosity and Perseverance, have tried to find answers to some of these questions. The answers are tantalizing, and we still don't know if Mars hosted life in the past or if it might still exist underground. Planetary scientists will continue to develop new Mars missions since the question of whether there is life beyond Earth is one of the biggest in science.
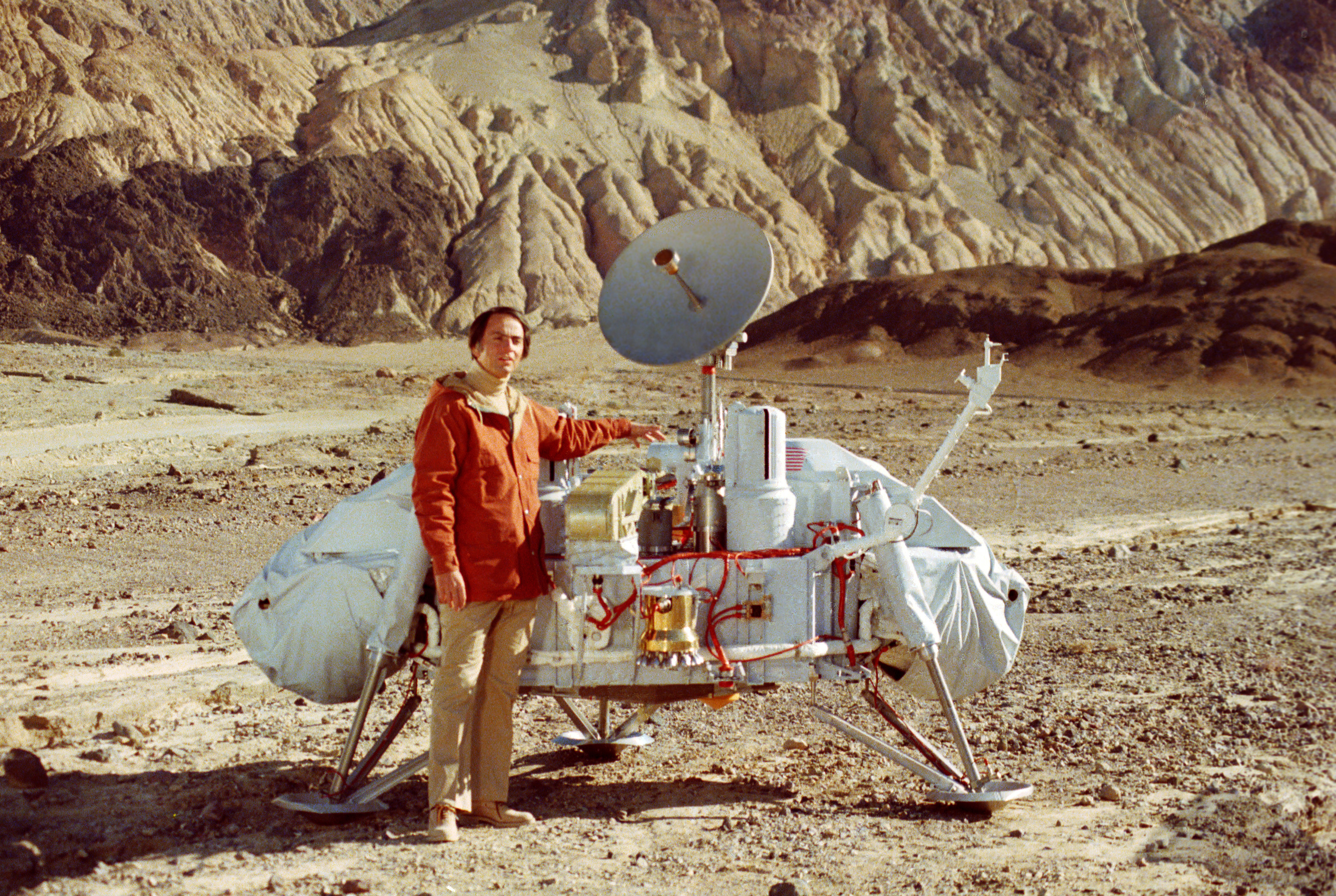
By the time the Viking landers were built in the 1970s, scientists suspected that Martian life might exist not as large organisms, but as microscopic bacteria or other simple organisms in the soil. To test this idea, both Viking landers contained equipment to make chemical tests of the soil. The first test looked for organic molecules in the soil. If there is life on Mars, then living or dead organisms should create a residue of organic molecules in the soil, as on Earth. None were found, to an accuracy of a few parts per billion. In other words, the Martian soil is sterile.
Such an emphatic result might seem to rule out any life on the planet. However, Viking scientists had another trick up their sleeves. They included three more experiments on each lander that scooped up soil, "fed" it nutrients, and watched for signs of metabolic activity (such as we see with plants growing and releasing carbon dioxide). All three experiments detected some unexpected chemical activity! However, careful analysis indicated it was probably not from living organisms, but from unusual chemical conditions in the soil that relate to an interesting fact: there is no ozone layer on Mars. This means that strong ultraviolet light from the Sun reaches the surface and destroys any exposed organic molecules. (Remember that the Earth is protected by its ozone layer.)
Ultraviolet radiation can thus explain the unusual results of both sets of experiments. On one hand, the UV would have destroyed any organics in the soils, potentially causing a false negative on experiments seeking evidence of life in the soil. On the other hand, this UV also creates unusual soil conditions that may help create the unusual oxides that caused the chemical reactions in the Viking soil experiments, thus creating a false positive. Put together, these results suggest that there is probably no life in the particular soils at the Viking sites, but this result is not entirely conclusive.
Some scientists have suggested that the soil within a meter or so of the surface might not be the best place to look for life on Mars. Annual storms stir up the surface dust and blow it around on Mars, effectively sterilizing the whole surface layer of the planet. A similar process occurs in Antarctica, where icy winds sterilize the soils. There, tiny life forms live not in the inhospitable soil, but in fractures inside rocks. The rocks offer an environment protected from the environmental extremes of the exposed surface. Thus, in the years after the Viking experiments, scientists speculated that life - or life's remnants - might be found hidden in subsurface layers or other protected environments of Mars as well. There is good indirect evidence for sub-surface aquifers on Mars, so liquid water may be present tens of meters under the surface, far deeper than any current or planned rover can dig.
The story of the search for life on Mars took an exciting turn in 1996 when we got to look inside a rock. NASA scientists in Houston were studying one of the oldest Martian meteorites, a Martian rock more than 4 billion years old. This rock had deposits of carbonates inside fractures in the rock, where liquid water had percolated. The carbonate contained concentrations of organic molecules as well as certain minerals that are produced on Earth by microbes that thrive in oxygen-poor environments. Were these features produced by non-biological chemical processes on Mars, or could they have been caused by forms of life?
The possible discovery of past life on Mars electrified the scientific community and it quickly became one of the biggest news stories of the year. Carl Sagan once said on the subject of life beyond Earth, "extraordinary claims require extraordinary evidence." Claims of UFOs as alien visitations are not supported by this level of evidence. How does the claim for ancient Martian life stack up? Most planetary scientists are unconvinced. The bottom line is a verdict of "not proven."
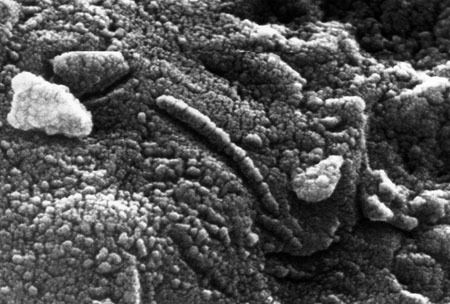
One issue is the origin of the Mars rock, called AHL84001. Although the odyssey of this rock from Mars to the Antarctic icecap seems extraordinary, we know that the gas trapped within the rock does not match the atmosphere of our planet but it is a perfect match for the gases sampled by the Viking landers. There is almost no doubt that the rock is from Mars. Another concern is the origin of the organic compounds in the rock. Perhaps they reflect contamination of the rock by terrestrial chemicals, which seeped in after the meteorite arrived on Earth. The chemicals are not generally concentrated toward the surface of the rock, as usually is the result of outside contamination. However, some of the organic material may represent terrestrial contamination. So it remains likely that some of the organic material came from Mars. There is also concern about the experimental methods used to study the meteorite.
The chemical evidence for life is unconvincing to many planetary scientists. The NASA researchers also found curious microscopic structures, and suggested that these may be fossils of actual Martian microbes. In the view of these researchers, microbial life may have evolved on Mars billions of years ago and perhaps died out later or persisted in primitive form in hidden sites on Mars. Other teams have found organic concentrations in at least one other Martian meteorite. It sounds good, so why do many planetary scientists consider the evidence for ancient Martian life still controversial? The answer is that the chemical evidence does not point uniquely to life.
Part of the concern stems from the difficulty of interpreting the chemical evidence. There are many complex chemical reactions that do not involve living organisms. So it is difficult to prove that chemical traces were caused by a living metabolism. Another concern is the interpretation of the "fossil" structures. They are much smaller than normal terrestrial bacteria, although they are similar in size to bacteria that exist in underground or nutrient-poor environments on Earth. Yet the evidence is ambiguous. The picture does not show the telltale signs of cell walls. Moreover, some scientists have argued that traces like these "fossil" forms are left when a rock is subject to extreme forces and sudden heating — which must have occurred when the rock was blasted off the surface of Mars.
The issue of looking for life on Mars illustrates how science works. While trying to unravel the mystery of AHL84001, there are conclusions we can reach with near certainty (the fact that the rock is from Mars), and conclusions we can not reach with much certainty at all (the fact that microbial life existed in the rock). Most of the key evidence — the chemical traces, the "fossil" forms — has more than one possible explanation. Other future tests will involve better chemical and isotopic analyses of the composition of the organic molecules. These tests might show more conclusively whether or not the chemical traces were created by living matter. Many research groups are now working with fragments of the few Mars rocks we have. We may never be sure until we go back to Mars for more evidence.
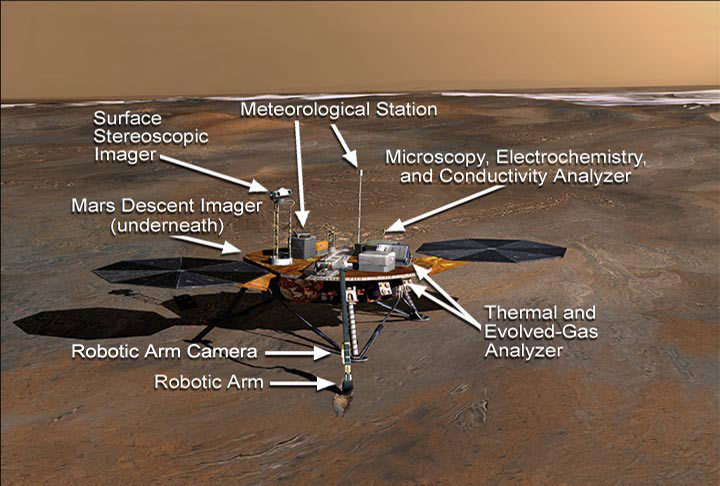
As if to egg us on and encourage our return, Mars keeps offering us new and inconclusive data. In 2008, the Mars Phoenix lander found evidence of water ice on Mars - something needed for life as we understand it on Earth. In 2009, methane was found in the Martian atmosphere. In general, methane only comes from either volcanic/geologic activity (not known to exist on Mars) or from life (on Earth, cows are one of the biggest producers of methane!). Even though the methane is at a very low level, a few parts per billion, it varies over time which is hard to understand if it has a purely geological origin.
What has humanity learned about the question of life on Mars? First, the theories of a century ago have been ruled out. There are no Martian civilizations, nor are there any large animals or plants. Second, although biological evolution has produced incredible adaptations to a range of conditions on Earth — from the dark, cold sea floor to hot geothermal pools in Yellowstone Park — it apparently was not able to evolve advanced forms under the surface conditions on Mars. On the other hand, during the more moist early history of Mars, biochemical evolution may have produced microbial Martian life, which may have died out since then. The most exciting possibility is that life could still exist in a primitive form in water that exists under pressure just under the Martian surface. Most of the research on Mars over the next few decades will be aimed at confirming or refuting this hypothesis. If it is confirmed, we will have exciting proof that life can evolve on other worlds, and that intelligent life on Earth may not be unique.
In the late 1980s, President George Bush announced an ambitious goal for NASA to send humans to Mars in the next few decades. The Soviet Union, too, with its large space station, seemed to be inching toward human exploration of Mars. However, these plans were never well funded, and they languished with the collapse of the Soviet Union. In the 1990s, President Clinton determined that no major effort to land humans on Mars would be pursued for the next decade. Sending astronauts to Mars would be a formidable and dangerous adventure, costing hundreds of billions of dollars. Compared to the Moon voyages, it is hundreds of times further to travel and would take years instead of weeks. Robotic space probes might not capture the public imagination the way an astronaut does, but they are cheaper and safer.
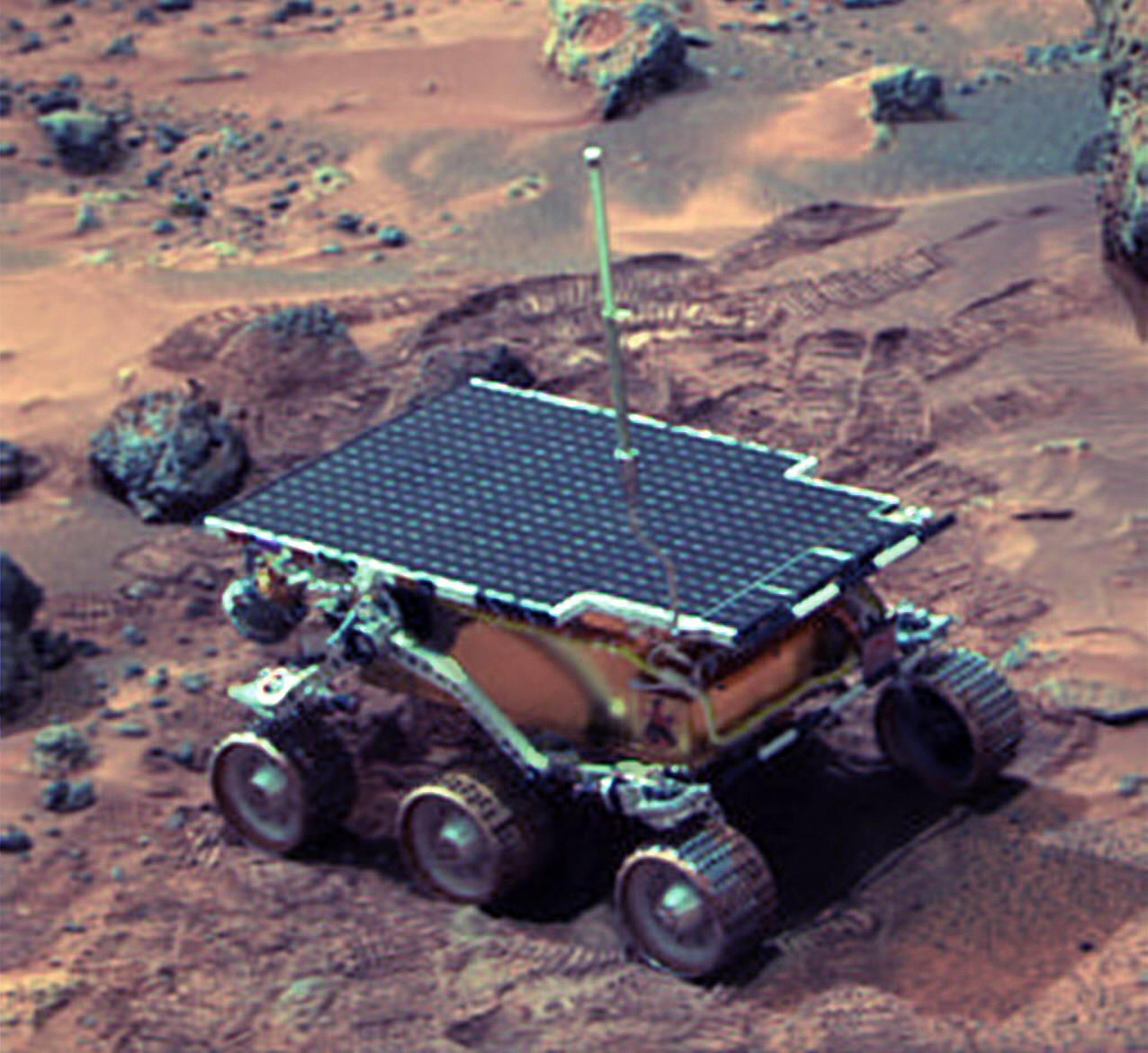
Therefore, the U.S. is pursuing vigorous plans to send various unmanned probes to Mars in the next decade. These missions, with various international partners, will send cameras, spectrometers, and geochemical instruments to gain more data about the climate history of Mars. They will follow on from the spectacular success of the relatively cheap Mars Pathfinder, which sent a small vehicle roaming over the surface of Mars in 1997. NASA's program was hampered by several expensive failures in 1999. Russia also planned major Mars missions, but the launch failure of its international Mars probe in 1996 devastated the nation's financially strapped planetary program. Several more missions are proposed by the U.S., Europe, and Russia in the next decade to set up a network of weather and seismic instruments to monitor Martian conditions. An attempt to return rock and soil samples is still being discussed, with a Mars sample return mission scheduled by NASA in about a decade and a possible human landing a few years after that.